How to Find Happiness With LiFePO4 (Lithium-Ion) Batteries
By: Rob Beckers
You have just sold your first-born into slavery, remortgaged the house, and bought yourself a lithium-ion battery! Now you want to know how to maintain your precious new purchase: How to best charge lithium-iron-phosphate batteries, how to discharge them, and how to get the maximum life out of your lithium batteries. Luckily LiFePO4 batteries are easy to take care of! This article will explain the do’s and don’ts to extend their lifespan.
Table Of Contents
- Why Lithium-Ion?
- Battery Co$t of Lithium-Ion vs. Lead-Acid
- Battery Bank Sizing for LFP
- But Wait a Minute!
- Other BMS-Induced Peculiarities & Problems
- Electrical Code & Lithium Batteries
- How Does a LiFePO4 Battery Work?
- Charging an LFP Battery
- Discharging an LFP Battery
- Storing Lithium-Ion Batteries
- The End of Your Lithium-Ion Batteries
- Take-Home Lessons
Pricing of lithium-ion batteries is slowly changing from obscenely expensive to only moderately unaffordable, and we at Solacity are seeing a steady increase in sales of this type of battery. At this point, the vast majority grid-tie hybrid systems with battery backup are using lithium ion and a significant percentage of off-grid systems are installing them as well. Many users also put them to work in RVs, fifth-wheels, campers and similar vehicles.
This article will talk about one specific category of lithium-ion batteries; Lithium-Iron-Phosphate or LiFePO4 in its chemical formula, also abbreviated as LFP batteries. These are a little different from what you have in your cell phone and laptop, those are (mostly) lithium-cobalt batteries. The advantage of LFP is that it is much more stable, and not prone to self-combustion. That does not mean the battery cannot combust in case of damage: There is a whole lot of energy stored in a charged battery and in case of an unplanned discharge the results can get very interesting very quickly! LFP also lasts longer in comparison to lithium-cobalt, and is more temperature-stable. Of all the various lithium battery technologies out there this makes LFP best suited for deep-cycle applications!
Since this seems to be a cause of confusion for some: All Lithium-Iron-Phosphate batteries are in fact lithium-ion batteries, but not all lithium-ion batteries are Lithium-Iron-Phosphate batteries. The term “lithium-ion battery” is a generic description of any battery technology that uses lithium ions to move and store electrical charge. Many chemistries use lithium ions to do this, and LiFePO4 is one such specific lithium-ion chemistry.
We will assume the battery has a BMS or Battery Management System, as almost all LFP batteries that are sold as a 12/24/48 volt pack do. The BMS takes care of protecting the battery; it disconnects the battery when it is discharged, or threatens to be over-charged. The BMS also takes care of limiting the charge and discharge currents, monitors cell temperature (and curtails charge/discharge if needed), and most will balance the cells each time a full charge is done (think of balancing as bringing all the cells inside the battery pack to the same state-of-charge, similar to equalizing for a lead-acid battery). Unless you like living on the edge, DO NOT BUY a battery without BMS!
While this is a very good general rule to live by, there are exceptions worth noting: Some brands, Victron being one of them, make batteries that do not contain a built-in BMS. Instead, they provide an external BMS with all the hardware and instructions needed to wire the battery bank up safely. There is advantage to an external BMS (it can be replaced easily if it breaks), and this too provides a safe and useful solution.
What follows below is the knowledge gleamed from reading a large number of Web articles, blog pages, scientific publications, and discussion with LFP manufacturers. Be careful what you believe, there is a lot of disinformation out there! While what we write here is by no means meant as the ultimate guide to LFP batteries, our hope is that this article cuts through the bovine excrement and gives solid guidelines to get the most out of your lithium-ion batteries.
The Weak Link - The BMS
We now have a few years of experience with lithium-ion batteries and what is becoming clear is that while the LiFePO4 cells hold up very well, that is not the case with the Battery Management System (BMS). Overall the number of prematurely failed batteries is small, but with 10,000+ batteries sold it is clear that in 99% of cases it is the BMS that fails, turning the battery into an expensive piece of gender-neutral-cave decoration!
While we very much advocate for using batteries with a built-in BMS (without one, the battery would be unsafe and likely fail very quickly!), manufacturers struggle to make their BMS as bullet-proof as it should and needs to be. Surge currents due to the input capacitors of large inverters, motors, and air-conditioners can and at times will kill the BMS, rendering the battery useless.
At least one well-known battery manufacturer is now enforcing their warranty conditions to the letter, and that requires the use of an external current limiter when their batteries are used with large inverters (“large” being defined as 3,500 Watt and up). This leads to the ironical situation where the BMS is there to protect the battery, and now a current limiter gets connected to protect the BMS. What will be next to protect the current limiter…
Seeing how the BMS has become the weak link, manufacturers should really work (hard) on hardening that. Nothing is 100% bomb-proof, but there certainly is room for improvement! Another solution could be to acknowledge that the BMS is the weak link and make it so it can be replaced without too much effort, for example a gasketed lid on the battery that is removable with a few screws, and a BMS that has connectors and bolted lugs, so a repair shop can swap the board. And some battery manufacturers (*cough* Volthium *cough cough*) have started doing something like this for their batteries. It makes no sense to throw away a battery where 90% of the cost is in the cells, and 10% in the BMS, just because the BMS failed.
We explained in our lead-acid battery article how the Achilles heel of that chemistry is sitting at partial charge for too long. It is too easy to pooch an expensive lead-acid battery bank in mere months by letting it sit at partial charge. That is very different for LFP! You can let lithium-ion batteries sit at partial charge forever without damage. In fact, LFP prefers to sit at partial charge rather than being completely full or empty, and for longevity it is better to cycle the battery or to let it sit at partial charge.
But wait! There is more!
Lithium-ion batteries are very nearly the holy grail of batteries: With the right charge parameters you can almost forget there is a battery. There is no maintenance. The BMS will take care of it, and you can happily cycle away!
But wait! There is still more! (Any resemblance with certain infomercials is purely coincidental, and, frankly, we resent the suggestion!)…
LFP batteries can also last a very long time. Our Volthium LFP batteries are rated at 3500+ cycles, at a full 100% charge/discharge cycle. If you did that every day it makes for 9+ years of cycling! They last even longer when used in less-than-100% cycles, in fact for simplicity you can use a linear relationship: 50% discharge cycles means twice the cycles, 33% discharge cycles and you can reasonably expect three times the cycles. In reality you actually get more cycles than that, and very few real-life scenarios would see batteries drained a full 100% on a regular basis. For that reason we reasonably expect that most people will see their lithium batteries realistically last 20 years before needing replacements.
But wait! There is more yet!…
A LiFePO4 battery also weighs less than 1/2 of a lead-acid battery of similar capacity. It can handle large charge currents (100% of amp-hour rating is no problem, try that with lead-acid!), allowing for rapid charging, it is sealed so there are no fumes, and it has a very low self-discharge rate (3% a month or less).
As I am writing this, our WorldWide WWL31 100Ah 12 volt battery costs $727 Canadian dollars. The full 100Ah is certainly usable, so that makes 12 x 100 = 1,200 Watt-hour in energy storage, or $0.61 per Wh in usable energy storage.
One of our best-bang-for-the-deep-cycle-buck lead-acid batteries is the Rolls/Surrette S6 L16-HC (formerly S-550), currently going for $496 for 6V 445Ah in storage. With lead-acid only 80% is really reasonably useful, going into the bottom 20% of energy storage is a recipe for permanent battery damage, so we have 6 x 445 x 0.8 = 2,136 Wh in energy storage. That makes for 496 / 2136 = $0.23 per Wh in usable energy storage.
This is where you say, “wait a minute, those d@m$ lithium batteries are three times the price of lead-acid!!”. Immediately followed by “but I still really want one!”, and right you are: We did not figure the difference in battery life in yet.
The Surrette S6 L16-HC is good for around 1900 cycles at 50% Depth-Of-Discharge (DOD), while the Enerwatt will do 6000 cycles at that same 50% DOD. That means the lithium-ion battery is going to last about 3.2x as long! Over the life of a single set of LFP batteries the cost per usable Wh for lead-acid now works out to 3.2 x 0.23 = $0.74, exceeding the cost of lithium-ion! There is more to it than that: In real life very few people will get the full cycle life out of lead-acid batteries. It is too easy to have them take offence to your treatment and prematurely depart for the Big Battery in the Sky. If you do make them go the distance, there is watering, measuring specific gravity, and taking care to regularly recharge them lest they sulfphate. None of that is needed for lithium-ion!
We bet at this point you are willing to throw in that no-good spouse of yours (m/f, we don’t discriminate) with your first-born just so you can replace your lead-acid with lithium-ion batteries!
We hinted at this above: Lithium-ion batteries have 100% usable capacity, while lead-acid really ends at 80%. That means you can size an LFP battery bank smaller than a lead-acid bank, and still have it be functionally the same. The numbers suggest that LFP can be 80% the amp-hour size of lead-acid. There is more to this though.
For longevity lead-acid battery banks should not be sized where they regularly see discharging below 50% SOC. With LFP that is no problem! Round-trip energy efficiency for LFP is quite a bit better than lead-acid as well, meaning that less energy is needed to fill up the tank after a certain level of discharge. That results in faster recovery back to 100%, while we already had a smaller battery bank, reinforcing this effect even more.
The bottom line is that we would be comfortable to size a lithium-ion battery bank at 50% – 60% of the size of an equivalent lead-acid bank, and expect the same (or better!) performance. Including on those dark winter days when sun is in short supply.
Beware of Series Connected Batteries!
There is a potential issue when multiple lithium-ion batteries are connected in series. For example, two 12 volt 100 Ah batteries, each with their own built-in BMS, connected in series to make 24 volt 100 Ah. Now assume one of those two batteries is near-empty, the other pretty full, and you put a load on the batteries, to discharge them. The near-empty battery will reach the point where the BMS decides “enough is enough” first and it will switch off that battery, in effect disconnecting your entire battery bank, even though the other battery is still full.
The same potential for trouble exists when charging both batteries at the same time with a 24 volt charging source. The fuller of the two batteries will fill up first, raising the charging voltage over that battery, until reaching the point where the BMS once again intervenes to protect the battery and switches the full battery off. When the BMS switches off, your entire battery bank “goes away”. If both started off uneven, then the other battery may well be nowhere near full yet, and this will not resolve over time or multiple charge cycles either.
The moral of this story is that you should understand the dynamics of connecting multiple lithium-ion batteries in series. They do not quite behave like lead-acid batteries! Lead-acid batteries will self-balance when they are charged, all attaining a similar state-of-charge in the end. Lithium-ion batteries do not! From experience we know that by-and-large series connected batteries, each with their own BMS, can work fine. It would be a good idea though to make sure both are “in sync” every now and then, by charging them individually with a 12 volt charger, until both are known to be fully charged, so they start off with the same state-of-charge. Alternatively you can employ a battery-balancer to keep them in sync for you.
Because it is important to understand this, we will come back to it and other BMS-related peculiarities in more detail further below.
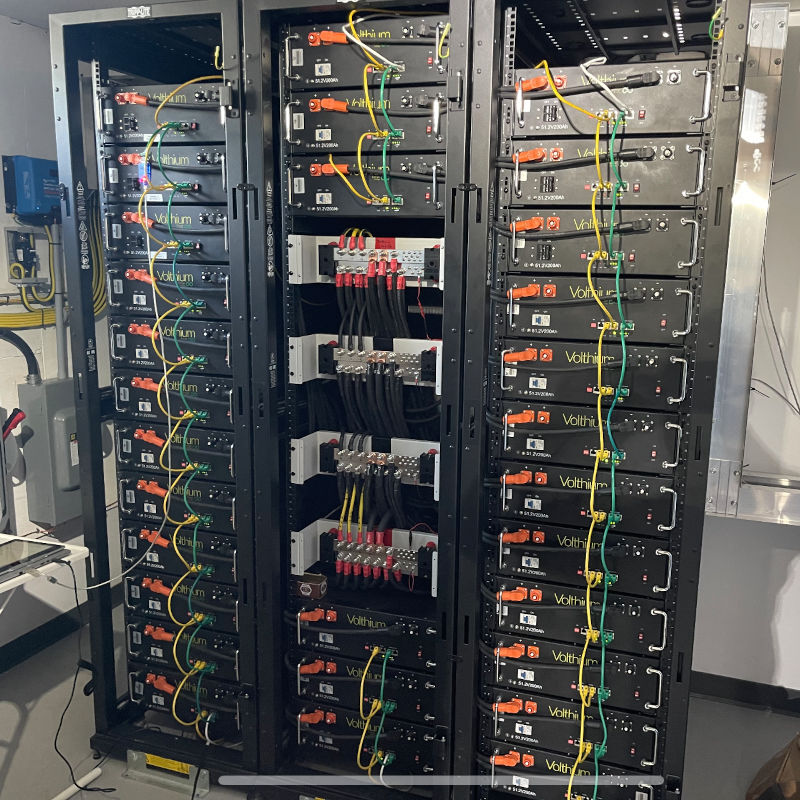
Is lithium-ion really the solution to all our battery woes? Well, not quite…
LFP batteries too have their limitations. A big one is temperature: You cannot charge a lithium-ion battery below freezing, or zero Centigrade. Lead-acid could not care less about this. You can still discharge the battery (at a temporary capacity loss), but charging is not going to happen. The BMS should take care to block charging at freezing temperatures, avoiding accidental damage. This is a Big Deal in our Canadian climate! Now, to be completely transparent, many lithium battery brands also make versions that have a built-in heater, so when they are charged below freezing the heater first heats up the cells. More about that in a moment.
Temperature is also an issue at the high end. The biggest single cause of aging of the batteries is use or even just storage at high temperatures. Up to around 30 Centigrade there is no problem. Even 45 Centigrade does not incur too much of a penalty. Anything higher really accelerates aging and ultimately the end of the battery though. This includes storing the battery when it is not being cycled. We will talk about this in more detail later, when discussing how LFP batteries fail.
There is a sneaky issue that can crop up when using charging sources that potentially provide a high voltage: When the battery is full the voltage will rise, unless the charging source stops charging. If it rises enough the BMS will protect the battery and disconnect it, leaving that charging source to rise even more! This can be an issue with (bad) car alternator voltage regulators, that need to always see a load or the voltage will spike and the diodes will release their magic smoke. This can also be an issue with small wind turbines that rely on the battery to keep them under control. They can run away when the battery disappears.
Then there is that steep, steep, initial purchase price!
But we bet you still want one!..
The BMS (Battery Management System) that is build into the batteries functions as a simple on-off switch, switching the batteries off when voltage, current, or temperature parameters get to the edge of what is safe. Contrary to what many think, the BMS does not change the charge or discharge current, it really is just an on-off switch; if you have a charging source that can push hundreds of amps into the battery the BMS will not prevent you from doing this, but it will sense the large current, and switch the battery off when the upper safe limit is reached!
Lithium Batteries with Built-In Heater
The issue of not being able to charge LiFePO4 batteries below freezing is now being overcome by versions that have a built-in heater and a thermostat that senses when the battery is being charged at a low temperature. It will kick in a heater to get the cells above freezing before actually charging the battery (incidentally this is also how an electrical car works in winter).
That may look like a good solution to the no-charging-below-freezing problem, but it too comes with its own potential issues: When the heater is running the battery cells are not charging (and vice-versa). This could cause problems when your charging sources can deliver more current than the heater needs, which in turn causes the ‘charge voltage’ (though note it’s not actually charging, just heating) to rise rapidly. Generally the charge controller will limit the voltage, and keep it from rising too high. However, this may erroneously cause the charge controller to believe it’s done bulk-absorb charging and go to its float stage before it is done heating, at which point the batteries will not actually be charged at all! The work-around is to create a custom charging profile for the charge controller, with a suggested value of 14.0 to 14.4 volt for bulk-absorb (for a 12V battery bank, multiply to fit your situation for 24/48V batteries), and 14.0 volt for float so it actually continues charging. Absorb time should still be kept low, 0.5 or 1 hour is suggested, and temperature compensation should be set to zero (or off). This way the batteries will get charged to at least 95% State-Of-Charge even when the heating cycle leaves the charge controller at float by the time it is done.
Because the battery cells are switched off while the battery heater is running, there is in essence no battery. That leaves the system with very little voltage-buffer, in other words a small current change causes a large voltage change, something that may not work with (for example) wind turbines that expect to see a large load to keep them under control.
If your system has a battery monitor that uses a shunt to measure the amp-hours going in, and coming out of the battery, this will lose track of the State-Of-Charge when a heated battery is involved. Any amp-hours going towards heating are not charging the battery, but they are counted by the battery monitor! There is no work-around for this, you will just not get good SOC readings from a shunt-based battery monitor when battery heating is involved.
Heated lithium-ion batteries should NEVER be connected in series! Unless the BMS’s in both batteries communicate, there is no way the heaters will be switched on/off at the exact same times, and that will cause all kinds of issues. So no series connection for heated LiFePO4 batteries.
The long and short of this is that you should think long and hard about heated LiFePO4 batteries. If at all possible it may be a better idea to stick with regular (unheated) ones, and use a well-insulated battery box to keep the temperatures above freezing.
Jump-Starting The BMS
For most conditions (over-current, over-voltage, under-temperature, or over-temperature) the BMS will automatically switch back on again, either after a set amount of time has passed, or once the conditions are safe. However, there is one case where the BMS will NOT switch on by itself, the battery will stay off: When any cell within a LFP battery falls below the lower safe voltage limit the BMS will switch off to protect the cells from over-discharge. It does this with still a little charge left in the cells, so the battery can sit for a while and self-discharge before damage to the cells occurs. The important part is that the BMS will not switch the battery back on by itself! When this happens the battery simply “goes away” and produces 0 volt.
To make the BMS switch on again after a low-voltage disconnect event the battery needs to see a charging voltage. How much exactly varies from brand-to-brand, but generally this means 14.0 volt or up (for a 12V battery). Keep in mind that inverter-chargers won’t work without a battery, nor will solar charge controllers. They need to see regular battery voltage to function. That means you cannot switch the battery BMS back on by charging from a generator (via your inverter-charger) or your solar panels. To make the BMS switch on again you would need a 120V AC charger that can do “dead battery charging” as it is usually called in the brochure, meaning it puts out a charging voltage even if it does not sense a battery. Alternatively you can “jump start” the switched-off battery by taking another battery of the same nominal voltage, even a lead-acid battery, and connect it in parallel with the dead battery, and then charge via solar or your inverter-charger. As soon as the voltage reaches high enough the BMS will sense it and switch the battery back on again. At that point you can disconnect the extra battery, but please keep charging so the empty battery does not immediately switch off again with the slightest load.
Balance & BMS
Another source of confusion, and potential problems, is when multiple single lithium-ion batteries, each with their own BMS, get connected in series to create a battery bank with a higher voltage. For example, by connecting four 12 volt batteries in series to create a 48V battery bank. Lithium-ion batteries do not at all self-balance! This is very different from lead-acid, where charging gets progressively less efficient as the battery gets more fully charged; this makes it so when multiple batteries are connected in series the ones that have less charge in them will automatically “catch up” to the fuller battery. Not so for lithium-ion batteries! Any difference in charge between series-connected batteries will persist from charge-cycle to charge-cycle and wreak havoc: Say a half-full 12V battery is connected to a fully charged 12V battery, in series, to create a 24V battery bank. When discharging the half-full battery will reach empty first, and at some point the BMS will intervene and switch that battery off, causing the entire battery bank to “go away”. Moreover, the empty battery will not switch on again until it sees a charging voltage. The second battery will at this point be about half full, and if the entire bank is charged in this state the situation will be exactly the same as before; one battery will reach full while the other is only just half-full. Worse still, the fully charged battery will continue to rise in voltage until the BMS intervenes and switches that battery off, causing the entire bank once again to just disappear (though it will switch back on again by itself, not needing a “jump start”). This situation will persist forever, unless manually corrected.
Victron Battery Balancer
Victron makes a product that can help balance multiple series-connected 12 volt lithium-ion batteries. Their Battery Balancer measures the voltage of each 12V battery during charging, and bypasses 1 amp of the battery with the higher voltage, to the battery with the lower voltage, until they are equal. It takes one Battery Balancer for a 24 volt battery bank, two for a 36 volt bank, and three for a 48 volt bank.
That means you HAVE to make sure that all batteries are at the same State-Of-Charge (SOC) before connecting lithium-ion batteries in series! The easiest way to ensure this is by fully 100% charging each battery before they are connected in series. For a set of 12V batteries that means (for example) using a 120V AC charger plugged into the grid or a generator, set it to an absorb voltage of 14.4V, and let it charge until no more current goes into the battery. Repeat this for each battery, and only then connect them series. Depending on how well they match, this may need to be repeated every now and then (once a year or so). Reports are encouraging that a group of series-connected batteries will continue to behave well over time, as long as they started out at the same SOC.
Note that this is only a factor for series-connected lithium-ion batteries. Parallel-connected batteries do not have this issue, they all see the same voltage and eventually will arrive at the same SOC.
It is normal for series connected lithium-ion batteries to each have a slightly different voltage (even if they are at the same SOC), and because the BMS will switch off batteries that exceed an upper safe value, it is a good idea to set any charging sources in the system to use the lower limit for the bulk-absorb voltage that will still fill up the battery. A good value would be 14.0 volt, with a 1-hour absorb time or less (on a 12V basis, multiply as needed). That will hopefully prevent any individual battery from rising above the upper cut-off limit. If this still causes the BMS of one or more batteries to intervene, try a lower absorb-time value such as 1/2 hour. If needed absorb time can be set all the way down to zero (or as low as settings allow), by the time a LiFePO4 battery reaches 14.0V it is already at least 95% full.
The bottom line is that when at all possible you should avoid connecting lithium-ion batteries in series! There now are 24V and 48V batteries that avoid the whole issue altogether. Our advise is to use those.
BMS Induced Power Limits
Unlike lead-acid batteries that can (briefly) deliver hundreds and even 1000+ amps in current, there is a hard limit to what the BMS in a lithium-ion battery will allow. There usually are several stages; this much for a fraction of a second, that much for a few seconds, and some lower limit for long periods of time. Exceeding those limits means the BMS will switch off, and the battery “goes away”. This affects the loads you can bolt onto a lithium-ion battery.
The typical 12 volt 100 Ah battery would, for example, have a limit of 100 amp continuous output current. That translates to (roughly) 12 x 100 = 1,200 Watt. Connect a 4,000 Watt inverter and you are guaranteed to never reach that level of output power! After 1,200 Watt the BMS will intervene and switch the battery off. It would take at least 4 of these batteries to be able to drive an inverter of this size and actually reach full continuous output!
Besides continuous output limits, there is a more insidious issue: Inverters have input capacitors on the battery side, to smooth out and handle surges in output power on the AC side. Large inverters have large input capacitors, and large capacitors cause very large currents to flow when they are connected to the battery. For large inverters, around 3 kW and up, this can easily reach in the hundreds of amps! While most lithium-ion batteries have a large limit for brief surges, this capacitor charge-up current can still exceed that limit, causing the BMS to switch the battery off. To make it possible to even connect these large inverters, the input capacitors need a chance to slowly charge up, and to do that a 4.7 kOhm 5 Watt resistor can be connected over a switch or breaker in line with the positive wire between battery bank and inverter. The resistor makes it so a little current flows into the inverter, slowly charging the capacitors, and by the time the switch or breaker is moved to the “on” position they are already charged up and there is no large current surge.
While it is important to understand the above in case problems arise, in practice we have found lithium-ion batteries to behave quite well. Even when connected in series. Just follow the guidelines we just talked about and you will likely be just fine!
Since lithium-ion batteries became a viable option for house-wide energy storage the Canadian Electrical Code (CEC) has been going nuts with new rules, making it more complicated with each new addition. Unfortunately it is not all that easy to comply! We will try and just highlight the main points, and since electrical code in Canada is provincial, this is mainly focused on Solacity’s province, Ontario (which closely follows the CEC). For those looking to pass electrical inspection our best advise is to sit down with your electrical inspector and go over the plans, even before buying any equipment!
The first wrinkle to wrap your head around is that electrical code sees lithium batteries no longer as ‘just’ a Battery Bank (CEC section 64-800), but an Energy Storage System (CEC section 64-900). Of course, they also expect a lithium battery bank to comply with everything in the Battery Bank section too! Each Canadian province adds their own flavour to the CEC, and for Ontario this is done in the form of ‘Bulletins’, in particular Bulletin 64-7-2 “Installation and Approval of Energy Storage Systems”, and Bulletin 64-8-2 “Battery based energy storage systems (ESS) at residential occupancies”. Other provinces tend to (eventually) follow Ontario when it comes to electrical code, so if yours is not quite as onerous yet, rejoice! And get your system installed pronto… Because it will soon follow.
There are a number of standards & certifications that apply to lithium-ion battery banks: UL9540, UL9540A, and UL1973 (note the absence of CSA-listing!). The reason these standards exist, and inspectors like them so much (and you should too!), is to prevent your house from burning down.
So what are these?
The Big One is UL9540. This is actually a combo: it certifies a particular lithium battery in combination with a particular inverter brand and type. At the time this is written UL9540 is the Holy Grail of certifications; installing a combo of battery and inverter that is UL9540-listed will give you that magic-carpet-like ride through electrical inspection! Note that our Volthium 48 volt rack-mount batteries are fully UL9540-listed with a number of inverters.
Then there is UL9540A. Sounds the same as UL9540, doesn’t it? Yet it is something utterly, totally different…
UL9540A is a cell-level test (actually not even a certification). A battery that lists UL9540A tells you the cells inside were subjected to UL9540A-testing, and there is a test report somewhere showing what sub-sections of the test the cells passed, and which ones they failed. UL9540A is not a pass-fail test or certification, all cells will pass some of the subsections, and fail others. It is most definitely NOT the same as UL9540 certification! Now, to meet UL9540 the cells inside the battery will have to meet most of the UL9540A tests, in particular they cannot self-combust (unlike your laptop and cell phone batteries!). Much of UL9540A is about thermal-runaway, and cells have to show that they do not spontaneously catch on fire when they get hot.
So what about lithium batteries that ‘claim’ UL9540A but do not have UL9540 (or do not have it with the inverter you plan on using)?
These may still pass electrical inspection, but there will be more hoops to jump through. It would be good if there are stickers on the batteries saying “This equipment meets the cell level performance criteria of UL9540A”, and “Suitable for use in residential dwellings where permitted”. This is not enough though, inspectors will still require that elusive CSA-sticker on them. Getting this will require what’s known as field inspection & approval, or CSA SPE-1000.
Lastly, UL1973 is just a fairly generic battery certification that ensures the battery meets mechanical, electrical, and BMS safety standards. Lithium-ion batteries will have to be certified for this one too, to stand a chance of passing UL9540 or SPE-1000.
Sometimes UL9540 Really Is NOT UL9540...
It has come to our attention that a number of our East Asian friends produce lithium-ion batteries that are stickered (and claim) full UL9540 compliance with a large number of inverter brands and types, when in reality they do not meet the rules in any way, shape, or form!
The way this scam works (and it is a scam, you are NOT buying a safe battery!) is that they first produce a battery with expensive cells that meet all the right UL9540A criteria, an expensive BMS to meet UL1973, and then get UL9540 for any and all inverters they can bring to the table. Once that’s done, and the battery goes into mass-production, the internals are changed: Cheap cells that were never subjected to UL9540A are substituted, and the BMS is replaced by one with far fewer, and far cheaper components. Only the outside still looks the same. These batteries are then sold on the US and Canadian markets at prices any of the real UL9540-battery brands cannot touch.
So if a battery deal looks too good to be true, maybe it is…
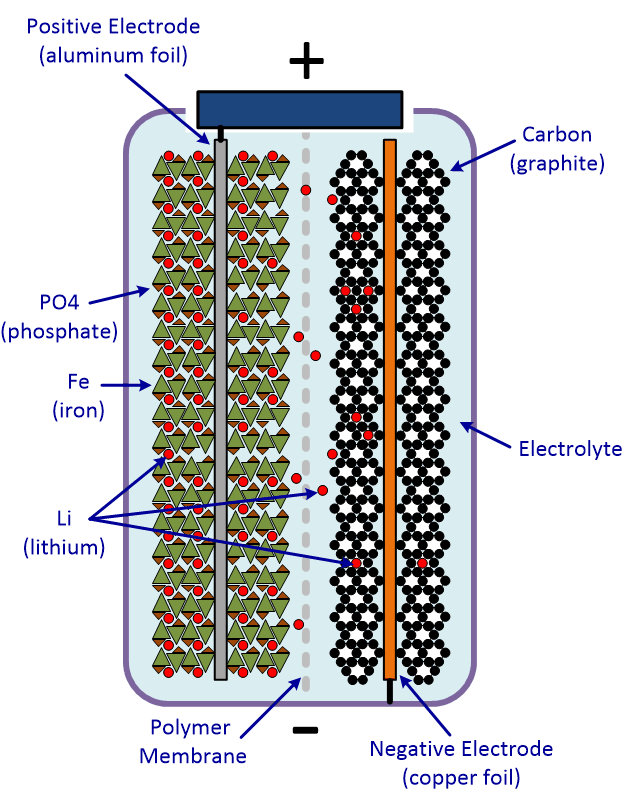
LiFePO4 Internal Structure
Lithium-ion batteries are referred to as a type of ‘rocking-chair’ battery: They move ions, in this case lithium ions, from the negative to the positive electrode when discharging, and back again when charging. The drawing on the right shows what is going on inside. The little red balls are the lithium ions, that move back and forth between the negative and positive electrodes.
On the left side is the positive electrode, constructed from lithium-iron-phosphate (LiFePO4). This should help explain the name of this type of battery! The iron and phosphate ions form a grid that loosely trap the lithium ions. When the cell is getting charged, those lithium ions get pulled through the membrane in the middle, to the negative electrode on the right. The membrane is made of a type of polymer (plastic), with lots of tiny little pores in it, making it easy for the lithium ions to pass through. On the negative side we find a lattice made of carbon atoms, that can trap and hold those lithium ions that cross over.
Discharging the battery does the same thing in reverse: As electrons flow away through the negative electrode, the lithium ions once again go on the move, through the membrane, back to the iron-phosphate lattice. They are once again stored on the positive side until the battery gets charged again.
If you have really been paying attention you now understand that the battery drawing shows an LFP battery that is almost completely discharged. Nearly all the lithium ions are on the side of the positive electrode. A fully charged battery would have those lithium ions all stored inside the carbon of the negative electrode.
In the real world lithium-ion cells are built of very thin layers of alternating aluminum – polymer – copper foils, with the chemicals pasted on them. Often they are rolled up like a jelly-roll, and put in a steel canister, much like an AA battery. The 12 volt lithium-ion batteries you buy are made of many of those cells, connected in series & parallel to increase the voltage and amp-hour capacity. Each cell is around 3.3 volt, so 4 of them in series makes 13.2 volt. That is just the right voltage for replacing a 12 volt lead-acid battery!
Most regular solar charge controllers have no trouble charging lithium-ion batteries. The voltages needed are very similar to those used for AGM batteries (a type of sealed lead-acid battery). The BMS keeps the battery safe, by making sure the battery cells see the right voltage, do not get overcharged, or overly-discharged, it balances the cells, and ensures the cell temperatures are within reason while they are being charged.
The graph below shows a typical profile of a LiFePO4 battery getting charged. To make it easier to read the voltages have been converted to what a 12 volt LFP battery pack would see (4x the single-cell voltage).
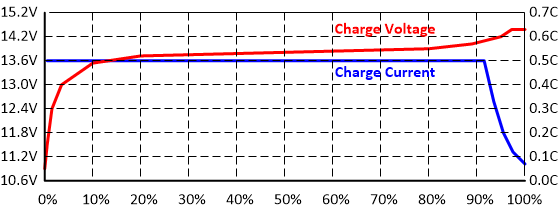
LiFePO4 Charge Voltage vs. SOC
Shown in the graph is a charge rate of 0.5C, or half of the Ah capacity, in other words for a 100Ah battery this would be a charge rate of 50 amp. The charge voltage (in red) will not really change much for higher or lower charge rates (in blue), LFP batteries have a very flat voltage curve.
Lithium-ion batteries are charged in two stages: First the current is kept constant, or with solar PV that generally means that we try and send as much current into the batteries as available from the sun. The voltage will slowly rise during this time, until it reaches the ‘absorb’ voltage, 14.4V in the graph above. As shown, once roughly 14.2V is reached the battery is already 95% full (in fact, 14.0V will get you over 90%), and to fill it the rest of the way the voltage is kept constant while the current slowly tapers off. Once the current drops to around 5% – 10% of the Ah rating of the battery it is at 100% State-Of-Charge.
In many ways a lithium-ion battery is easier to charge than a lead-acid battery: As long as the charge voltage is high enough to move ions it charges. Lithium-ion batteries do not care if they are not fully 100% charged, in fact they last longer if they are not. There is no sulphating, there is no equalizing, the absorb time does not really matter, you cannot really overcharge as would happen with lead-acid, just keep the charge voltage within reason, and the BMS will cut charging short if the voltage rises too much.
Charge Voltage Needed
So what voltage is enough to get those ions moving? A little experimenting shows that 13.6 volt (3.4V per cell) is the cut-off point; below that very little happens, while above that the battery will get at least 95% full given enough time. At 14.0 volt (3.5V per cell) the battery easily charges up to 90+ percent when it reaches that voltage, and an hour absorb time will fill it the rest of the way. For all intents and purposes there is little difference in charging between 14.0 or higher voltages, things just happen a little faster at 14.2 volt and above.
The current advise is to stay on the low side for the charging voltage, so 14.0 – 14.2 volt should work fine for most cases. This helps to not ‘overfill’ the battery and get better longevity out of it. Similarly, absorb time can be kept short. No absorb is needed for actually charging the battery, but many Battery Management Systems will use that last bit of charging (and absorb time) to balance the cells. For that reason 1 hour of absorb should work fine for most installs.
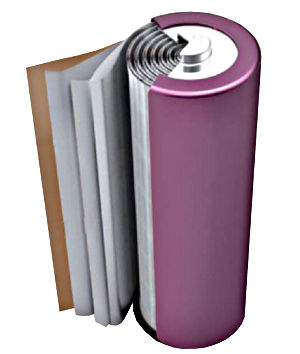
Lithium-ion cell structure
Bulk/Absorb Voltage
To summarize this, a bulk/absorb setting between 14.0 and 14.4 volt will work great for LiFePO4! Slightly higher voltages are possible, the BMS for most batteries will allow around 14.6 – 15.0 volt before disconnecting the battery. There is no benefit to a higher voltage though, just more risk of getting cut off by the BMS, and for longevity it is better to stay at the lower range of charge voltages.
Float Voltage
LFP batteries do not need to be floated. Charge controllers have this because lead-acid batteries have such a high rate of self-discharge that it makes sense to keep trickling in more charge to keep them happy. For lithium-ion batteries it is not great if the battery constantly sits at a high State-Of-Charge, so if your charge controller cannot disable float, just set it to a low enough voltage that no actual charging will happen. Any voltage of 13.6 volt or less will do.
If you live off-grid you may want to keep float voltage set at 13.6 volt though: This ensures that as long as the sun shines (assuming you have solar PV) your battery will be kept full. So you go into the night with (hopefully) full batteries.
Equalize Voltage
With charge voltages over 14.6 volt actively discouraged, it should be clear that no equalize should be done to a lithium-ion battery! If equalize cannot be disabled, set it to 14.6V or less (14.0 – 14.4V being an even better choice), so it becomes just a regular absorb charge cycle.
Absorb Time
There is a lot to be said for simply setting the absorb voltage to 14.0V or 14.2V, and then just stop charging once the battery reaches that voltage! In short, zero (or a short) absorb time. At that point your battery will be around 95% full. LiFePO4 batteries will be happier in the long run when they do not sit at 100% SOC for too long, so this practice will extend battery life. If you absolutely have to have 100% SOC in your battery then a little absorb will do that! Officially this is reached when the charge current drops to 5% – 10% of the Ah rating of the battery, so 5 – 10 amp for a 100Ah battery. If you cannot stop absorb based on current, then set absorb time to about 1 hour and call it a day.
Temperature Compensation
LiFePO4 batteries do not need temperature compensation! Please switch this off in your charge controller, or your charge voltage will be wildly off when it is very warm or cold.
Be sure to check your charge controller voltage settings against those actually measured with a good quality digital multi-meter! Small changes in voltage can have a big impact when charging a lithium-ion battery! Change the charge settings accordingly!
Unlike lead-acid batteries, the voltage of a lithium-ion battery stays very constant during discharge. That makes it difficult to divine the State-Of-Charge from voltage alone. For a battery with a moderate load the discharge curve looks as follows.
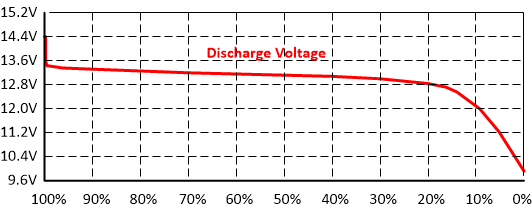
LiFePO4 Discharge Voltage vs. SOC
Most of the time during discharge, the battery voltage will be right around 13.2 volt. It varies by just 0.2 volt all the way from 99% to 30% SOC. Not long ago it was a Very Bad Idea™ to go below 20% SOC for a LiFePO4 battery. That has changed, and the current crop of LFP batteries will quite merrily discharge all the way down to 0% for many cycles. However, there is benefit in cycling less deep. Cycle life for lithium is not proportional: Cycling to 30% SOC will get you more than an extra 1/3 cycles vs. cycling down to 0%. Hard numbers are, well, hard to come by, but cycling down to 50% SOC seems to show around 3x the cycle life vs. cycling 100%.
Below is a table that shows battery voltage for a 12 volt battery pack vs. Depth-Of-Discharge. Take these voltage values with a grain of salt, the discharge curve is so flat that it really is hard to determine SOC from voltage alone. Small variations in load, and accuracy of the volt meter, will throw off the measurement.
State-Of-Charge | Voltage at rest (zero current) | Voltage under load (0.25C) |
---|---|---|
100% | 14.0 Volt | 13.6 Volt |
99% | 13.8 Volt | 13.4 Volt |
90% | 13.4 Volt | 13.3 Volt |
70% | 13.2 Volt | 13.2 Volt |
40% | 13.2 Volt | 13.1 Volt |
30% | 13.0 Volt | 13.0 Volt |
20% | 12.9 Volt | 12.9 Volt |
17% | 12.8 Volt | 12.8 Volt |
14% | 12.6 Volt | 12.5 Volt |
9% | 12.4 Volt | 12.0 Volt |
0% | 10.4 Volt | 10.0 Volt |
The very low self-discharge rate makes it easy to store LFP batteries, even for longer periods. It is no problem to put a lithium-ion battery away for a year, just make sure there is some charge in it before placing it in storage. Something between 50% – 60% is ideal, that will give the battery a very long time before self-discharge brings the voltage close to the danger point.
Storing batteries below freezing is fine, even at very low temperatures such as -40 Centigrade (that is the same in Fahrenheit), or even less! The electrolyte in LiFePO4 cells does not contain any water, so even when it freezes (which happens around -40 Centigrade, depending on the particular formulation) it does not expand, and does not damage the cells. Just let the battery warm up a bit before you start discharging it again, which is OK at -20 Centigrade and above. You will see an apparent loss of capacity when discharging at below-freezing temperatures that reverses when the battery gets above freezing, and there is a slightly accelerated effect on aging. Storing them at low temperatures is certainly much better than storage at high temperatures: Calendar aging slows down dramatically at low temperatures. Try to avoid storing them at 45 Centigrade and above, and try to avoid storing them completely full if possible (or nearly empty).
If you need to store batteries for longer periods, be sure to simply disconnect all wires from them. That way there can not be any stray loads that slowly discharge the batteries.
We hear you gasp in horror; the thought of your precious LFP battery bank departing the earthly realm surely sends shivers down your spine! Alas, all good things eventually must come to an end. What we want to prevent is an end of the premature (and possibly spectacular) kind, and to do that we have to understand how lithium-ion batteries die.
Battery manufacturers consider a battery “dead” when its capacity falls to 80% of what it should be. So, for a 100Ah battery, its end comes when its capacity is down to 80Ah. There are two mechanisms at work towards the demise of your battery: Cycling and aging. Each time you discharge and recharge the battery it does a little bit of damage, and you lose a little bit of capacity. But even if you put your precious battery in a beautiful glass-enclosed shrine, never to be cycled, it will still come to an end. That last one is called calendar life.
It is difficult to find hard data on calendar life for LiFePO4 batteries, very little is out there. Some scientific studies were done on the effect of extremes (in temperature, and SOC) on calendar life, and those help set limits. What we gather is that if you do not abuse your battery bank, avoid extremes, and generally just use your batteries within reasonable bounds, there is an upper limit of around 20 years on calendar life.
Besides the cells inside the battery, there is also the BMS, which is made out of electronic parts. When the BMS fails, so will your battery. So far empirical evidence shows that the BMS is more likely to die before the cells. Hopefully that will change (or at least change so the BMS can be swapped out when needed).
Processes inside the battery conspire over time to coat the boundary layer between electrodes and electrolyte with chemical compounds that prevent the lithium ions from entering and leaving the electrodes. Processes also bind lithium ions into new chemical compounds, so they are no longer available to move from electrode to electrode. Those processes will happen no matter what we do, but they are very much dependent on temperature! Keep your batteries under 30 Centigrade and they are very slow. Go over 45 Centigrade and things speed up considerably! Public enemy no. 1 for lithium-ion batteries, by far, is heat!
There is more to calendar life and how quickly a LiFePO4 battery will age: State-Of-Charge has something to do with it as well. While high temperatures are bad, these batteries really, really do not like to sit at 0% SOC and very high temperatures! Also bad, though not quite as bad as 0% SOC, is for them to sit at 100% SOC and high temperatures. Very low temperatures have less of an effect. As we discussed, you cannot (and the BMS will not let you) charge LFP batteries below freezing. As it turns out, discharging them below freezing, while possible, does have an accelerated effect on aging as well. Nowhere near as bad as letting your battery sit at a high temperature, but if you are going to subject your battery to freezing temperatures it is better to do so while it is neither charging nor discharging, and with some gas in the tank (though not a full tank). In a more general sense, it is better to put away these batteries at around 50% – 60% SOC if they need longer-term storage.
If you really want to know, what happens when a lithium-ion battery gets charged below freezing is that metallic lithium is deposited on the negative (carbon) electrode. Not in a nice way either, it grows in sharp, needle-like structures called ‘dendrites’, that eventually puncture the membrane and short out the battery (leading to a spectacular Rapid Unscheduled Disassembly Event as NASA calls it, involving smoke, extreme heat, and quite possibly flames as well). Lucky for us, this is something the BMS prevents from happening.
We are moving on to cycle life. It has become common to get thousands of cycles, even at a full 100% charge-discharge cycle, out of lithium-ion batteries. There are some things you can do though to maximize cycle life.
We talked about how LiFePO4 batteries work: They move lithium ions between the electrodes. It is important to understand that these are actual, physical particles, that have a size to them. They are yanked out of one electrode and stuffed into the other, each time you charge-discharge the battery. This causes damage, in particular to the carbon of the negative electrode. Each time the battery gets charged the electrode swells a bit, and each discharge it slims down again. Over time that causes microscopic cracks. It is because of this that charging to a little below 100% will give you more cycles, as will discharging to a little above 0%. Also, think of those ions as exerting “pressure”, and extreme State-Of-Charge numbers exert more pressure, causing chemical reactions that are not to the benefit of the battery. That is why LFP batteries do not like to be put away at 100% SOC, or put into float-charging at (near) 100% for long periods of time.
How fast those lithium ions get yanked hither and yon has an effect on cycle life as well. In light of the above that should be no surprise. While LFP batteries will routinely do charging and discharging at 1C (i.e. 100 amp for a 100Ah battery), you will see more cycles out of your battery if you limit this to more reasonable values. Lead-acid batteries have a limit of around 20% of Ah rating, and staying within this for lithium-ion will have benefits for a longer battery life as well.
The last factor worth mentioning is voltage, though this is really what the BMS is designed to keep in check. Lithium-ion batteries have a narrow voltage window, for both charging and discharging. Going outside that window very quickly results in permanent damage, and on the high end a possible RUD Event (NASA-talk, as mentioned before). For LiFePO4 that window is about 8.0V (2.0V per cell) to 16.8 volt (4.2V per cell). The built-in BMS should take care to keep the battery well within those limits.
Now that we know how lithium-ion batteries work, what they like and dislike, and how they ultimately fail, there are some pointers to take away. We have made a little list below. If you are going to do nothing else, please take note of the first two, they have by far the most effect on the overall time you will get to enjoy your lithium-ion battery! Taking heed of the others will help too, to make your battery last even longer.
To sum up, for long and happy LFP battery life, in order of importance, you should be mindful of the following:
- Keep the battery temperature under 45 Centigrade (under 30C if possible) – This is by far the most important!!
- Keep charge and discharge currents under 0.5C (0.2C preferred)
- Keep battery temperature above 0 Centigrade when discharging if possible – This, and everything below, is nowhere near as important as the first two
- Do not cycle below 10% – 15% SOC unless you really need to
- Do not float the battery at 100% SOC for long periods of time if possible
- Do not charge to 100% SOC if you do not need it
- KISS (Keep It Simple Smart-person) – avoid series-connected lithium batteries, avoid heated batteries unless absolutely needed
That is it!
Now you too can find happiness and a fulfilling life with your LiFePO4 batteries!